VEGF: Inflammation and Tumourigenesis
What is VEGF?
Vascular Endothelial Growth Factors (VEGFs) are a group of homodimeric polypeptides, and are master regulators of vascular development, maintenance and angiogenesis. VEGF is also a potent growth factor, and is produced by macrophages and CD4+ and CD8+ T cells. Members of the VEGF family include 5 structurally related proteins (VEGFA-D, and placental growth factor (PIGF), which are further individually subdivided based on alternative splicing. VEGF acts as a potent stimulator of angiogenesis, promoting the growth and survival of endothelial cells, and is essential for embryonic development and tissue repair in adults.
The discovery of VEGF and its subsequent characterization have significantly advanced our understanding of angiogenesis and its implications in various physiological and pathological conditions. VEGF-mediated angiogenesis is vital for the development of new blood vessels during embryonic development, wound healing, and tissue regeneration. Additionally, VEGF has been implicated in numerous diseases, including cancer, diabetic retinopathy, and age-related macular degeneration
Structure and Function of VEGF
VEGF is a glycoprotein that exists in different isoforms, resulting from alternative splicing of the VEGF gene. The major isoforms of VEGF are VEGF-A, VEGF-B, VEGF-C, VEGF-D, and PlGF. VEGF-A, in particular, is the most extensively studied isoform and is primarily responsible for promoting angiogenesis. Different isoforms of VEGF exhibit variations in their biological activities and binding affinity to specific receptors.
Upon binding to its receptors—VEGFR-1 (also known as Flt-1) and VEGFR-2 (also known as KDR or Flk-1)—VEGF initiates intracellular signaling cascades that regulate endothelial cell proliferation, migration, survival, and permeability. Activation of VEGFR-2 is mainly responsible for mediating angiogenic signaling, while VEGFR-1 can modulate VEGF activity and vessel stability.
In addition to angiogenesis, VEGF also influences other processes, such as vasculogenesis (the formation of new blood vessels during embryonic development), lymphangiogenesis (formation of lymphatic vessels), and the maintenance of vascular homeostasis. VEGF's multifunctional nature highlights its significance in various physiological and pathological contexts.
VEGF Signaling
VEGF is upregulated in response to hypoxic and metabolic perturbations, such as dysregulated glucose levels, primarily by the activation of the transcription factor NF-kB. VEGFs bind with high affinity to one of the three tyrosine kinase receptors; VEGFR1, VEGFR2, and VEGFR3, which are expressed highly on endothelial cells and monocytes. It has also been demonstrated that VEGF can bind to several non-VEGF receptors, such as neuropilin receptors (NRP), and heparin sulphate peptidoglycans (HSPG), which act as co-receptors and facilitate complex downstream signalling. Canonical VEGF signalling occurs mostly between a homodimeric VEGF molecule with a homodimeric VEGF receptor, and is induced upon ligation of VEGF to its membrane bound receptor VEGFR, leading to autophosphorylation of the cytoplasmic domains on the receptor and subsequent downstream signalling, initiated by the binding of adaptor molecules to the tyrosine residues of the VEGFR.
VEGF signaling involves the activation of VEGFR-1 and VEGFR-2, receptors found primarily on endothelial cells. Upon ligand binding, VEGFR-2 dimerizes and autophosphorylates, initiating downstream signaling events. These include the activation of PLC-γ, leading to calcium release and protein kinase C (PKC) activation. PI3K is also recruited, generating PIP3 to activate Akt, promoting cell survival, proliferation, and migration. Src family kinases are involved in cell adhesion and migration. VEGF signaling orchestrates endothelial cell responses crucial for angiogenesis and vascular development. It also affects other cell types, impacting processes like tumor progression and inflammation.
VEGF Signaling Pathway
Canonical and Non-canonical VEGF Receptor Signaling
Canonical VEGF receptor signaling involves well-established pathways, including activation of PLC-γ, leading to calcium release and PKC activation. It also recruits PI3K, activating Akt, and involves Src family kinases for cell adhesion and migration.
Non-canonical VEGF receptor signaling, although less understood, contributes to diverse cellular responses. For example, VEGFR-1 can induce cell migration and modulate immune cell recruitment, while VEGFR-2 can activate p38 MAPK, impacting endothelial cell migration and vessel sprouting.
Regulation of VEGF Expression
VEGF expression is tightly regulated under normal physiological conditions. Hypoxia-inducible factor 1 (HIF-1) is a key regulator of VEGF expression. In response to low oxygen levels, HIF-1 activates the transcription of VEGF by binding to specific regions in the VEGF gene promoter. This mechanism allows cells to adapt to hypoxic conditions by promoting angiogenesis.
Apart from hypoxia, other factors can also modulate VEGF expression. Growth factors, such as epidermal growth factor (EGF), fibroblast growth factor (FGF), and insulin-like growth factor (IGF), have been shown to induce VEGF expression in various cell types. Additionally, inflammatory cytokines, including tumor necrosis factor-alpha (TNF-α) and interleukin-1 beta (IL-1β), can stimulate VEGF production.
VEGF Related Kits
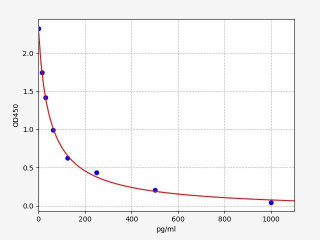
Human ANG 1-7 / Angiotensin 1-7 ELISA Kit | |
---|---|
ELISA Type | Sandwich |
Sensitivity | 9.375pg/ml |
Range | 15.625-1000pg/ml |

Human CD105 / Endoglin ELISA Kit | |
---|---|
ELISA Type | Sandwich |
Sensitivity | 0.094ng/ml |
Range | 0.156-10ng/ml |
The role of VEGF in disease
VEGF is a proliferative and vascular inducing molecule and plays a vital role in the development and maintenance of tumours. Tumour-infiltrating lymphocytes (TILs) can induce the upregulation of VEGF, thereby promoting vascularity in the tumour microenvironment and promoting tumourigenesis, as VEGF can induce further polarization of T cells to the Th1 subset. Moreover, autocrine VEGF signalling has generated a lot of interest in the triple negative breast cancer field, with a focus on VEGF-integrin signalling crosstalk. Additionally, VEGF is of key importance in the central nervous system, and mediates neuroprotection and neurogenesis, with VEGF levels dramatically decreased upon aging. The role of VEGF in the CNS is somewhat polarized, as VEGF can induce blood-brain barrier leakage upon injury, stroke and multiple neurodegenerative diseases. Furthermore, ligand-independent activation of VEFR2 contributes to the pathogenesis of diabetes, via upregulated reactive oxygen species.
VEGF as a therapeutic target
The main anti-cancer target in VEGF signalling is VEGFA-VEGFR2 association, which has long been shown to inhibit tumour growth. Multiple drugs have been developed to target this interaction. Bevacizumab, a major breakthrough in anti-VEGF therapeutics, is a monoclonal antibody which neutralizes VEGFA and has been approved for use in treatments for colon cancer, glioblastoma and breast cancer. Several other molecules targeting this pathway include VEGFR neutralizing antibodies, soluble VEGFR peptides, and an anti-PIGF antibody. Anti-VEGF therapy has also been demonstrated to improve the pathogenesis of rheumatoid arthritis, due to the resulting decreased levels of IL-6. VEGF is also an attractive target for pro-angiogenic therapy, such as following cardiac ischaemia and traumatic brain injuries, in order to regenerate cells.
Written by Lauryn McLoughlin
Lauryn McLoughlin completed her undergraduate degree in Neuroscience before completing her masters in Biotechnology at University College Dublin.
Recent Posts
-
Exhibition at the University of Oxford's BioEscalator
Showcasing Innovation Assay Genie recently took part in an engaging event at Oxfo …10th Jul 2025 -
Department of Oncology Retreat 2025
Department of Oncology RetreatAssay Genie was honoured to sponsor and participate in t …30th May 2025 -
Cure CLCN4 2025
Cure CLCN4 2025Assay Genie was honored to attend and present at the 2025 Cure CLCN4 Sc …26th May 2025